Motor vs. sensory predictions
Chapter 4 endnote 50, from How Emotions are Made: The Secret Life of the Brain by Lisa Feldman Barrett.
Some context is:
...these regions predict that your heart rate should increase and your blood vessels should dilate, for instance, in preparation to run. A pounding heart and surging blood would cause interoceptive sensations, so your brain must predict those sensations as well.
The brain's control of the body, sent as body-budgeting predictions, and interoceptive predictions come from the same columns of neurons and are related information. (They may even be exactly the same pattern of neural firing, just arriving at different places.) To the neurons in subcortical regions and in the spinal cord, the information is a body-budgeting prediction to the body (Figure B, below), whereas to the sensory regions, it’s a sensory prediction (Figure D). This arrangement is sometimes called corollary discharge or sending efference copies.
Neurons in Layers V and VI of body-budgeting (visceromotor) regions in the brain (which are part of the interoceptive network; see blue regions in Figure A) send predictions to Layers II through III to posterior/mid insula, which serves as primary interoceptive cortex. This serves to change the firing of those neurons in advance of incoming thalamic input from the body to Layer IV of primary interoceptive cortex, completing the simulated sensation.[1]
Body-budgeting regions send a variety of efference copies to motor cortex (Figure C) and to other sensory cortices (Figure D).
Figures
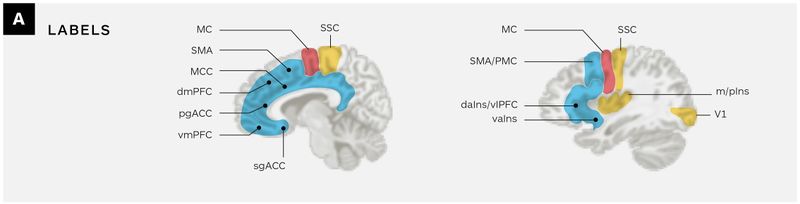
Legend: daIns = dorsal anterior insula (and in this figure includes ventrolateral prefrontal cortex); dmPFC = dorsomedial prefrontal cortex; m/pIns = mid/posterior insula (primary interoceptive cortex); MC = motor cortex; MCC = midcingulate cortex; pgACC = pregenual anterior cingulate cortex; PMA = premotor area; sgACC = subgenual anterior cingulate cortex; SMA = supplementary motor area; SSC = somatosensory cortex; V1 = primary visual cortex; vaIns = ventral anterior insula; vmPFC = ventromedial prefrontal cortex.
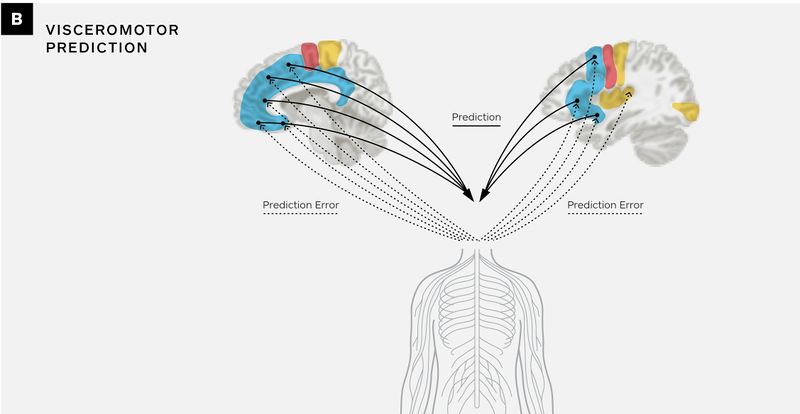
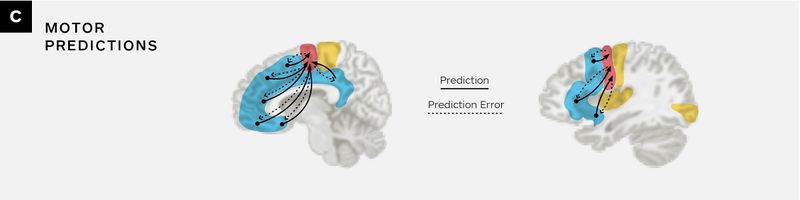
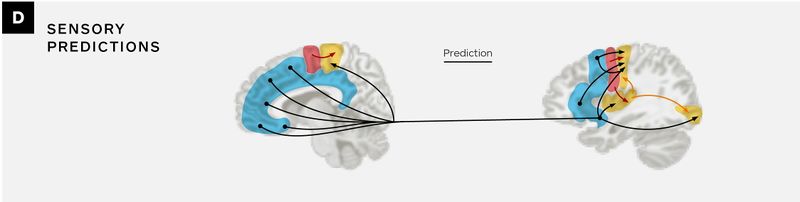
Notes on the Notes
- ↑ As explained in Barrett, Lisa Feldman, and W. Kyle Simmons. 2015. “Interoceptive Predictions in the Brain.” Nature Reviews Neuroscience 16 (7): 419–429.
- ↑ 2.0 2.1 As explained in Barrett, Lisa Feldman. 2017. "The theory of constructed emotion: an active inference account of interoception and categorization." Social Cognitive and Affective Neuroscience 12 (1): 1-23.
- ↑ As explained in Kleckner, Ian, Jiahe Zhang, Alexandra Touroutoglou, Lorena Chanes, Chenjie Xia, W. Kyle Simmons, Karen Quigley, Bradford Dickerson, and Lisa Feldman Barrett. 2017. “Evidence for a Large-Scale Brain System Supporting Interoception in Humans.” Nature Human Behavior 1: 0069.
- ↑ Bastos, Andre M., W. Martin Usrey, Rick A. Adams, George R. Mangun, Pascal Fries, and Karl J. Friston. 2012. "Canonical microcircuits for predictive coding." Neuron 76 (4): 695-711.
- ↑ Adams, Rick A., Stewart Shipp, and Karl J. Friston. 2013. "Predictions not commands: active inference in the motor system." Brain Structure and Function 218 (3): 611-643.
- ↑ Barrett, Lisa Feldman, and W. Kyle Simmons. 2015. “Interoceptive Predictions in the Brain.” Nature Reviews Neuroscience 16 (7): 419–429.
- ↑ Chanes, Lorena, and Lisa Feldman Barrett. 2016. “Redefining the Role of Limbic Areas in Cortical Processing.” Trends in Cognitive Sciences 20 (2): 96–106.
- ↑ For evidence that primary motor cortex is granular in structure, see Barbas, Helen, and Miguel Á. García-Cabezas. 2015. "Motor cortex layer 4: less is more." Trends in Neurosciences 38 (5): 259-261.